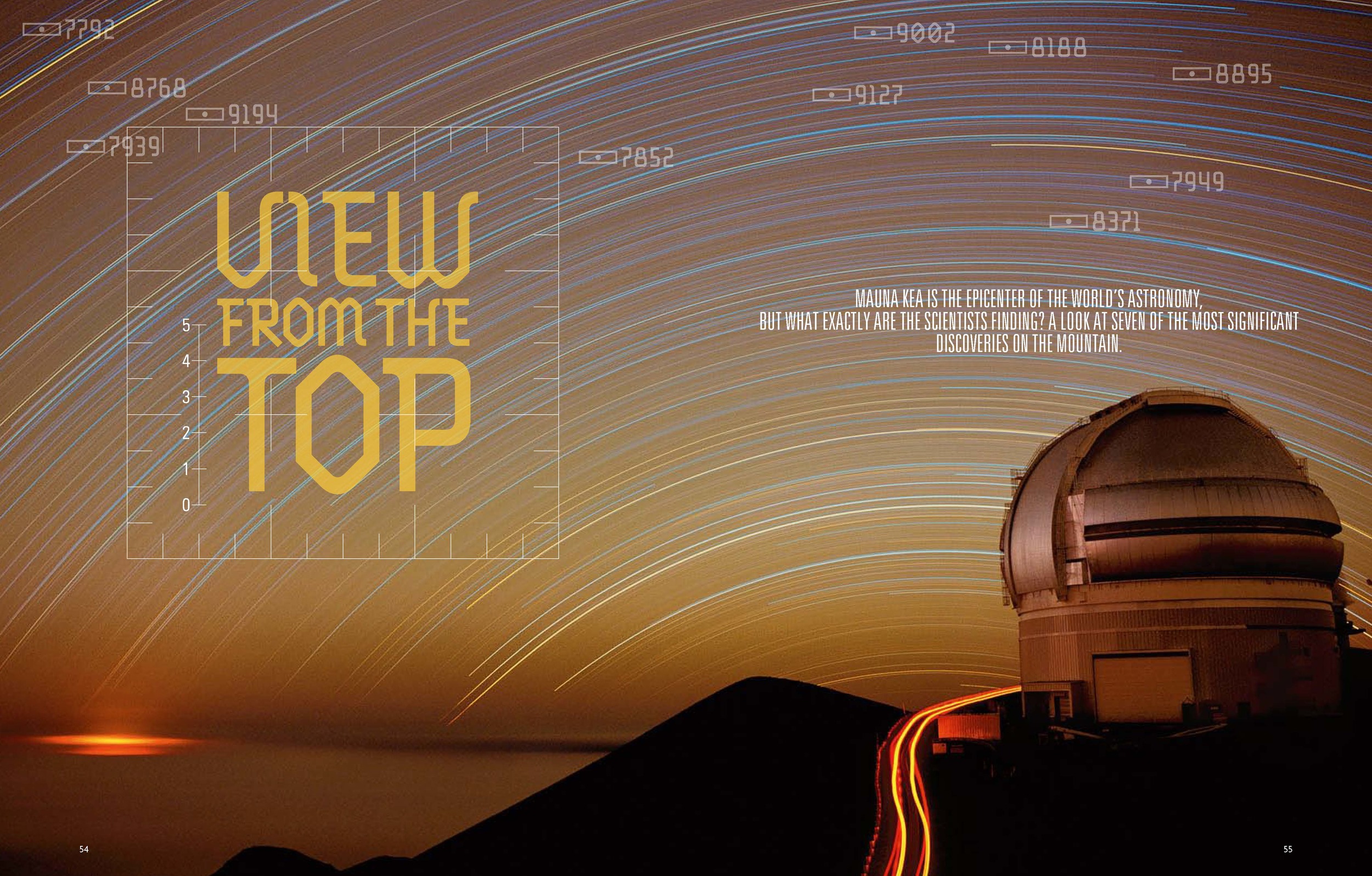
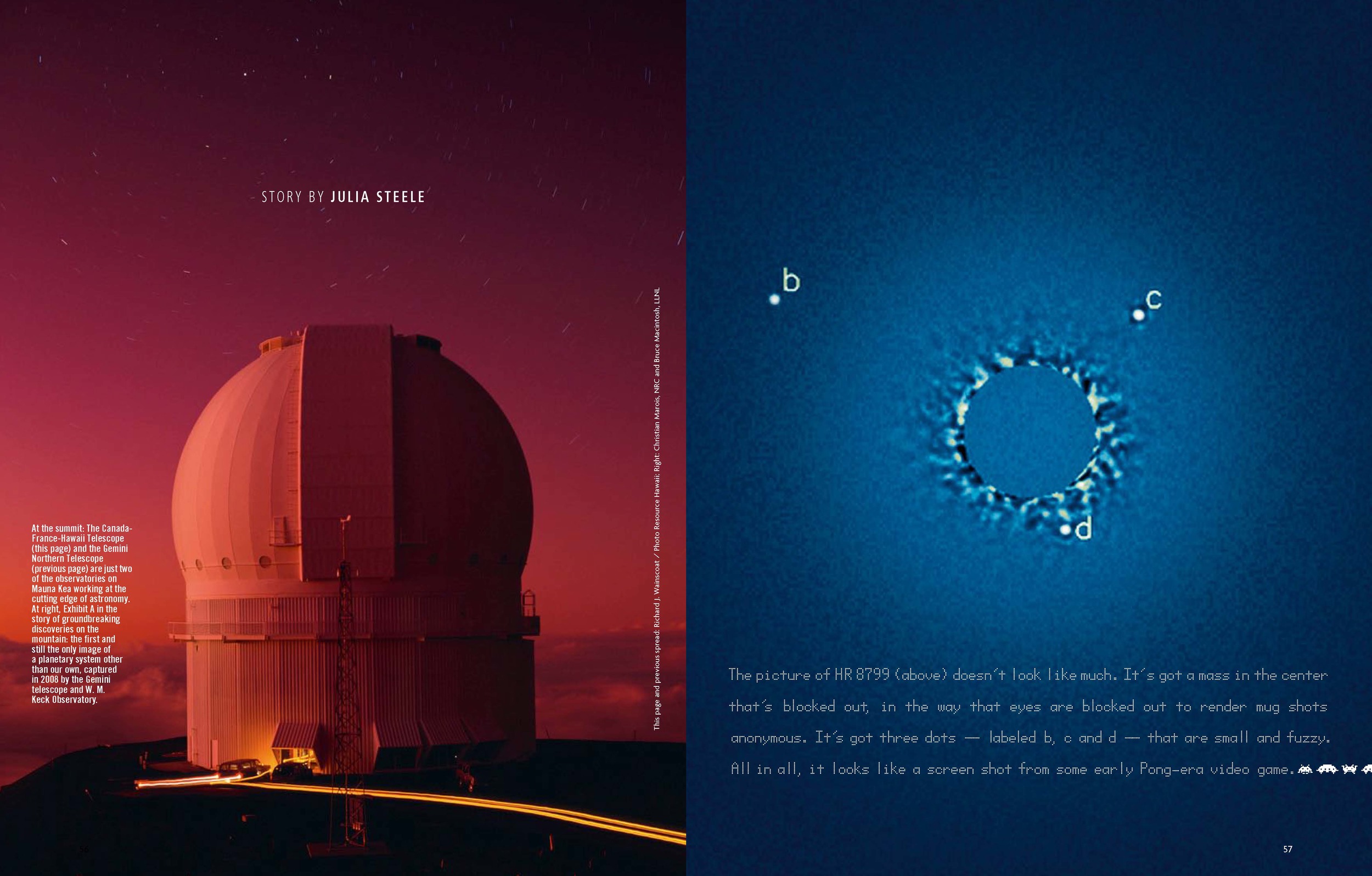
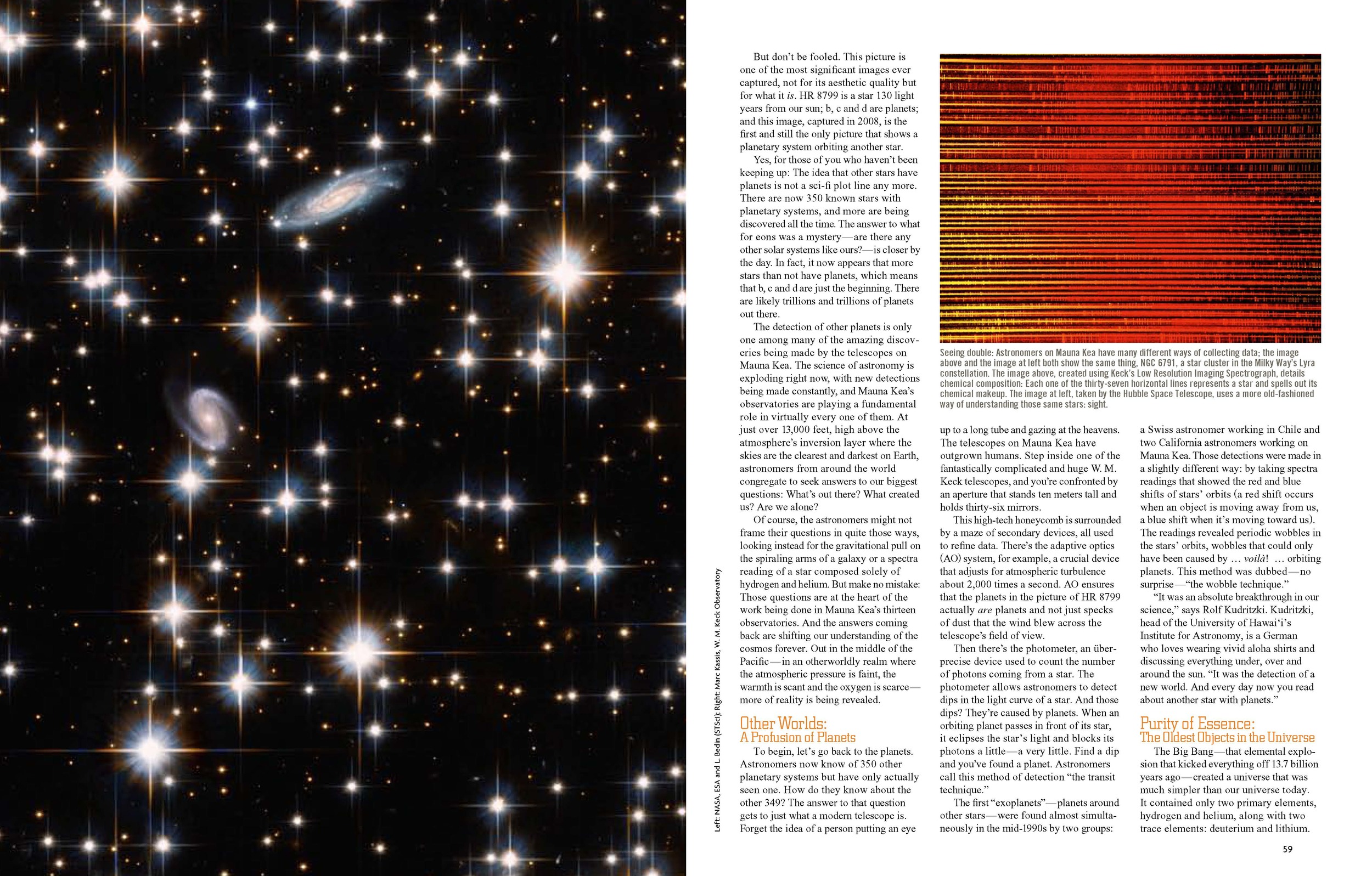
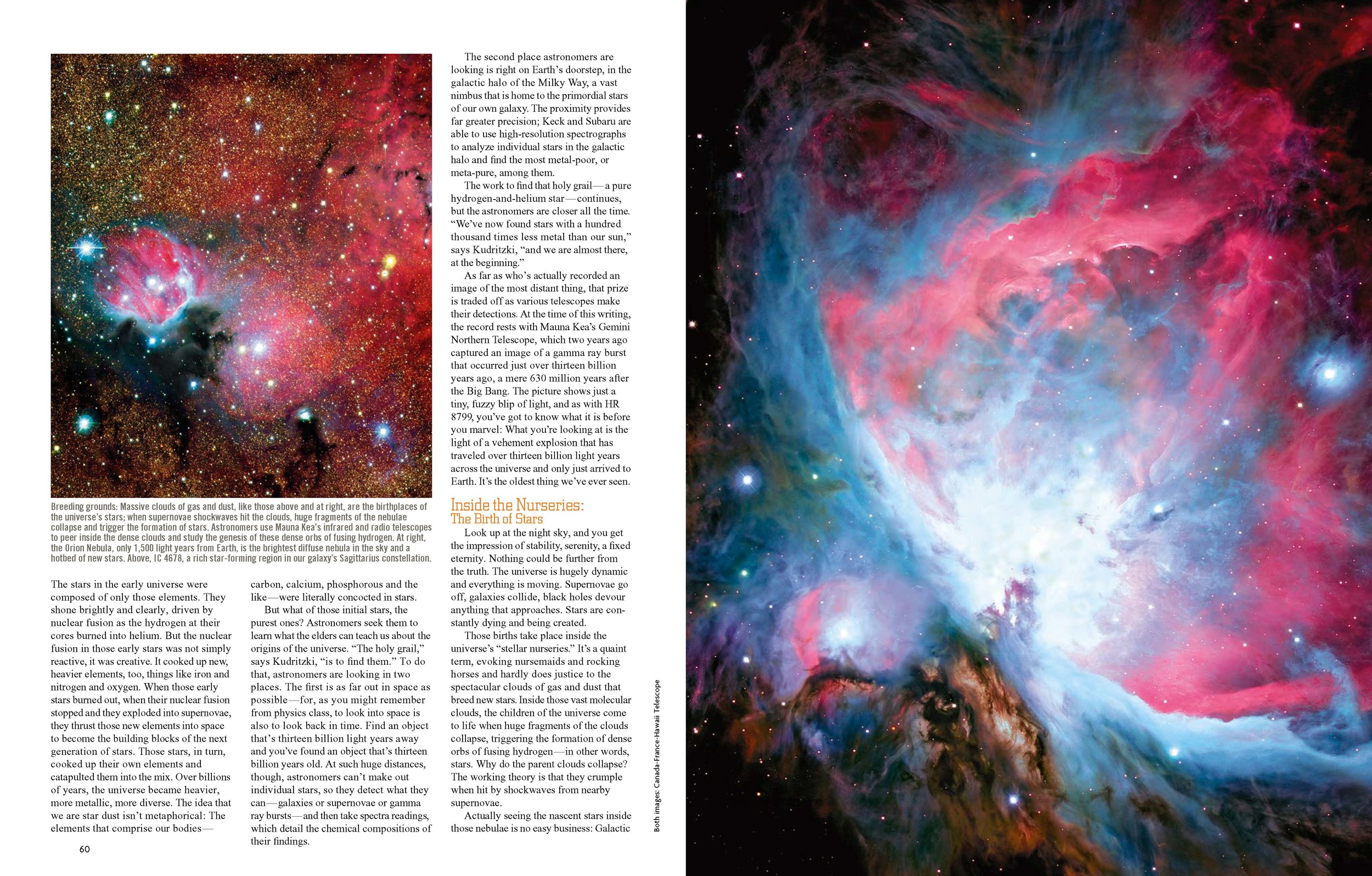
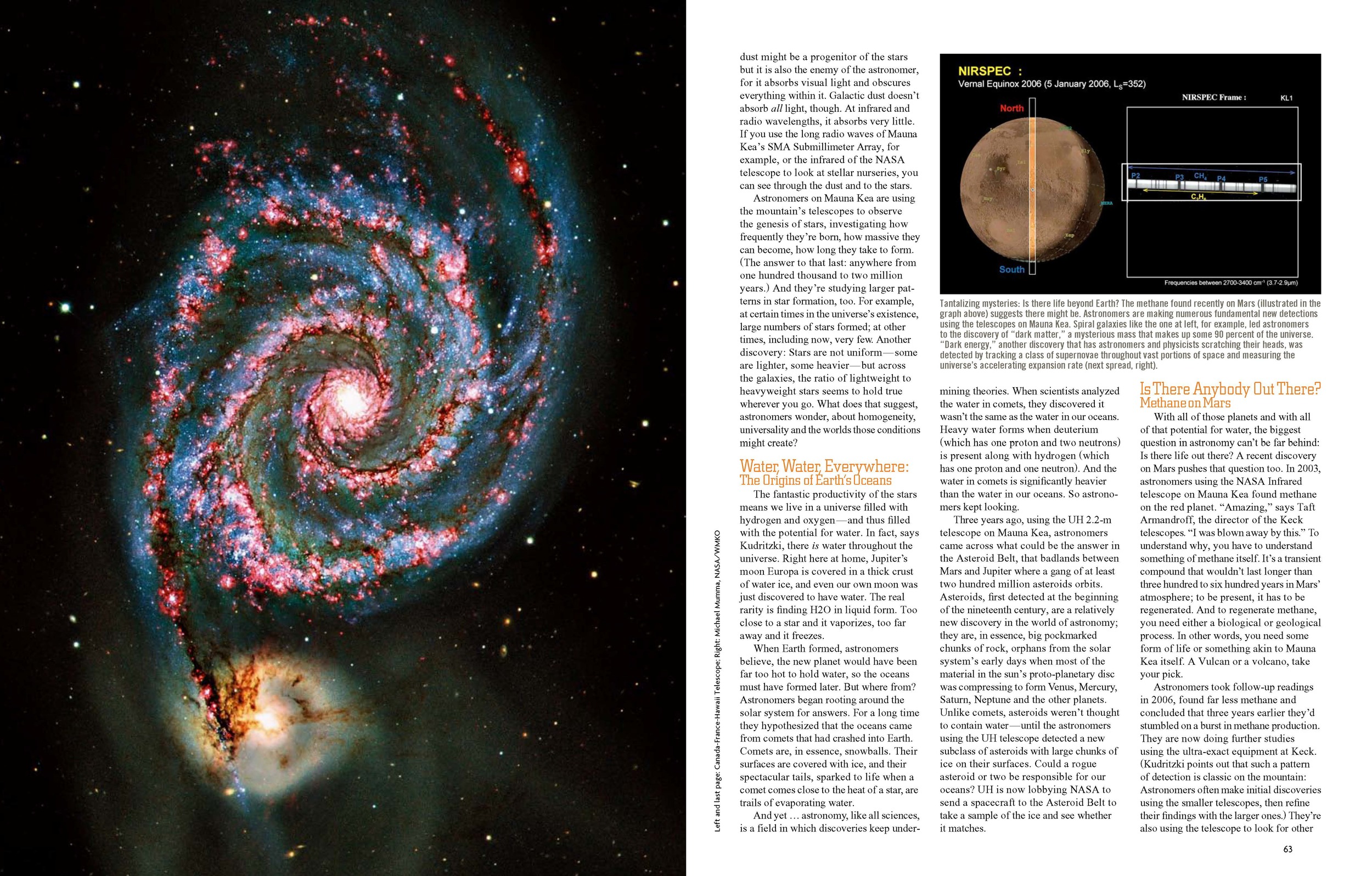
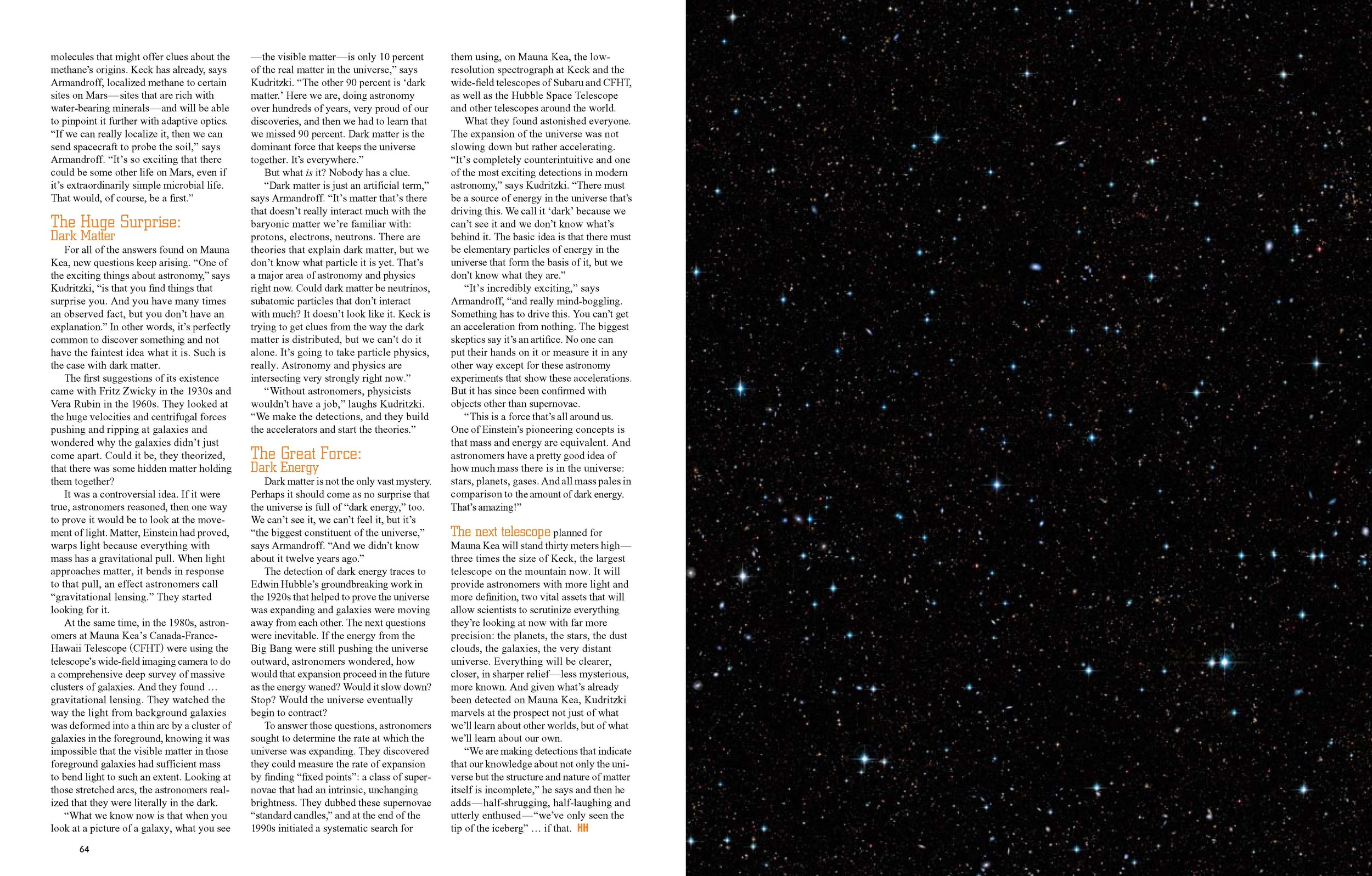
The picture of HR 8799 doesn’t look like much. The mass at its center is blocked out, in the way that eyes are blocked out to render mug shots anonymous. Around that center are three dots—labeled b, c and d—that are small and fuzzy. All in all, the picture looks like a screen shot from some early video game. But don’t be fooled. This picture is one of the most significant images ever captured, not for its aesthetic quality, but for what it is. HR 8799 is a star 130 light years from our sun; b, c and d are planets; and this image, captured in 2008, is the first and still the only picture that shows a planetary system orbiting another star.
Yes, the idea that other stars have planets is not a sci-fi plot line any more. There are now 350 known stars with planetary systems, and more are being discovered all the time. The answer to what for eons was a mystery—are there any other solar systems like ours?—is closer by the day. In fact, it now appears that more stars than not have planets, which means that b, c and d are just the beginning. There are likely trillions and trillions of planets out there.
The detection of other planets is only one among many of the amazing discoveries being made in the telescopes on Mauna Kea. The science of astronomy is exploding right now, with new detections being made constantly, and Mauna Kea’s observatories are playing a fundamental role in virtually every one of them. At just over 13,000 feet, high above the atmosphere’s inversion layer where the skies are the clearest and darkest on earth, astronomers from around the world congregate to seek answers to our biggest questions: What’s out there? What created us? Are we alone?
Of course, the astronomers might not frame their questions in quite those ways, looking instead for the gravitational pull on the spiraling arms of a galaxy or a spectra reading of a star composed solely of hydrogen and helium. But make no mistake: Those questions are at the heart of the work being done in Mauna Kea’s thirteen observatories. And the answers coming back are shifting our understanding of the cosmos forever. Out in the middle of the Pacific—in an otherworldly realm where the atmospheric pressure is faint, the warmth is scant and the oxygen is scarce—more of the cosmos is being revealed.
Other Worlds: A Profusion of Planets
To begin, let’s go back to the planets. Astronomers now know of 350 other planetary systems but have only actually seen one. How do they know about the other 349 and counting? The answer to that question gets to just what a modern telescope is. Forget the idea of a person putting an eye up to a long tube and gazing at the heavens. The telescopes on Mauna Kea have outgrown humans. Step inside one of the fantastically complicated and huge W.M. Keck telescopes, and you’re confronted by an aperture that stands ten meters tall and holds thirty-six mirrors.
This high-tech honeycomb is surrounded by a maze of secondary devices, all used to refine data. There’s the adaptive optics (AO) system, for example, a crucial device that adjusts for atmospheric turbulence about 2,000 times a second. AO ensures that the planets in the picture of HR 8799 actually are planets and not just specks of dust that the wind blew across the telescope’s field of view.
Then there’s the photometer, an über-precise device used to count the number of photons coming from a star. The photometer allows astronomers to detect dips in the light curve of a star. And those dips? They’re caused by planets. When an orbiting planet passes in front of its star, it eclipses the star’s light and blocks its photons a little—a very little. Find a dip and you’ve found a planet. Astronomers call this method of detection “the transit technique.”
The first “exoplanets”—planets around other stars—were found almost simultaneously in the mid-1990s by two groups: a Swiss astronomer working in Chile and two California astronomers working on Mauna Kea. Those detections were made in a slightly different way: by taking spectra readings that showed the red and blue shifts of stars’ orbits (a red shift occurs when an object is moving away from us, a blue shift when it’s moving toward us). The readings revealed periodic wobbles in the stars’ orbits, wobbles that could only have been caused by … voilà! … orbiting planets. This method was dubbed the “wobble technique.”
“It was an absolute breakthrough in our science,” says Rolf Kudritzki. Kudritzki, head of the University of Hawai‘i’s Institute for Astronomy, is a German who loves wearing vivid aloha shirts and discussing everything under, over and around the sun. “It was the detection of a new world. And every day now you read about another star with planets.”
Purity of Essence: The Oldest Objects in the Universe
The Big Bang—that elemental explosion that kicked everything off 13.7 billion years ago—created a universe that was much simpler than our universe today. It contained only two primary elements, hydrogen and helium, along with two trace elements: deuterium and lithium. The stars in the early universe were composed of only those elements. They shone brightly and clearly, their energy driven by nuclear fusion as the hydrogen at their cores burned into helium. But the nuclear fusion in those early stars was not simply reactive, it was creative. It cooked up new, heavier elements, too, things like iron and nitrogen and oxygen. When those early stars burned out, when their nuclear fusion stopped and they exploded into supernovae, they thrust those new elements into space to become the building blocks of the next generation of stars. Those stars, in turn, cooked up their own elements and catapulted them into the mix. Over billions of years, the universe became heavier, more metallic, more diverse. The idea that we are star dust isn’t metaphorical: The elements that comprise our bodies—carbon, calcium, phosphorous and the like—were literally concocted in stars.
But what of those initial stars, the purest ones? Astronomers seek them to learn what the elders can teach us about the origins of the universe. “The Holy Grail,” says Kudritzki, “is to find them.” To do that, astronomers are looking in two places. The first is as far out in space as possible—for, as you might remember from physics class, to look into space is also to look back in time. Find an object that’s thirteen billion light years away and you’ve found an object that’s thirteen billion years old. At such huge distances, though, astronomers can’t make out individual stars, so they detect what they can—galaxies or supernovae or gamma ray bursts—and then take spectra readings, which detail the chemical compositions of their findings.
The second place astronomers are looking is right on Earth’s doorstep, in the galactic halo of the Milky Way, a vast nimbus that is home to the primordial stars of our own galaxy. The proximity provides far greater precision; Keck and Subaru are able to use high-resolution spectrographs to analyze individual stars in the galactic halo and find the most metal-poor, or meta-pure, among them. The work to find that hHoly gGrail—a pure hydrogen-and-helium star—continues, but the astronomers are closer all the time. “We’ve now found stars with a hundred thousand times less metal than our sun,” says Kudritzki, “and we are almost there, at the beginning.”
As far as who’s actually recorded an image of the most distant thing, that prize is traded off as various telescopes make their detections. At the time of this writing, the record rests with Mauna Kea’s Gemini Northern Telescope, which two years ago captured an image of a gamma ray burst that occurred just over thirteen billion years ago, a mere 630 million years after the Big Bang. The picture shows just a tiny, fuzzy blip of light, and as with HR 8799, you’ve got to know what it is before you marvel: What you’re looking at is the light of a vehement explosion that has traveled over thirteen billion light years across the universe and only just arrived to Earth. It’s the oldest thing we’ve ever seen.
Inside the Nurseries: The Birth of Stars
Look up at the night sky, and you get the impression of stability, serenity, a fixed eternity. Nothing could be further from the truth. The universe is hugely dynamic and everything is moving. Supernovae go off, galaxies collide, black holes devour anything that approaches. Stars are constantly dying and being created.
Those births take place inside the universe’s “stellar nurseries.” It’s a quaint term, evoking nursemaids and rocking horses, and hardly does justice to the spectacular clouds of gas and dust that breed new stars. Inside those vast molecular clouds, the children of the universe come to life when huge fragments of the clouds collapse, triggering the formation of dense orbs of fusing hydrogen—in other words, stars. Why do the parent clouds collapse? The working theory is that they crumple when hit by shockwaves from nearby supernovae.
Actually seeing the nascent stars inside those nebulae is no easy business: Galactic dust might be a progenitor of the stars but it is also the enemy of the astronomer, for it absorbs visual light and obscures everything within it. Galactic dust doesn’t absorb all light, though. At infrared and radio wavelengths, it absorbs very little. If you use the long radio waves of Mauna Kea’s SMA Submillimeter Array, for example, or the infrared of the NASA telescope to look at stellar nurseries, you can see through the dust and to the stars.
Astronomers on Mauna Kea are using the mountain’s telescopes to observe the genesis of stars, investigating how frequently they’re born, how massive they can become, how long they take to form. (The answer to that last: Anywhere from one hundred thousand to two million years.) And they’re studying larger patterns in star formation, too. For example, at certain times in the universe’s existence, large numbers of stars formed; at other times, including now, very few. Another discovery: Stars are not uniform—some are lighter, some heavier—but across the galaxies, the ratio of lightweight to heavyweight stars seems to hold true wherever you go. What does that suggest, astronomers wonder, about homogeneity, universality and the worlds those conditions might create?
Water, Water, Everywhere: The Origins of Earth’s Oceans
The fantastic productivity of the stars means we live in a universe filled with hydrogen and oxygen—and thus filled with the potential for water. In fact, says Kudritzki, there is water throughout the universe. Right here at home, Jupiter’s moon Europa is covered in a thick crust of ice, and even our own moon was just discovered to have water. The real rarity is finding H2O in liquid form. Too close to a star and it vaporizes, too far away and it freezes.
When Earth formed, astronomers believe, the new planet would have been far too hot to hold water, so the oceans must have formed later. But where from? Astronomers began rooting around the solar system for answers. For a long time they hypothesized that the oceans came from comets that had crashed into Earth. Comets are, in essence, snowballs. Their surfaces are covered with ice, and their spectacular tails, sparked to life when a comet comes close to the heat of a star, are trails of evaporating water.
And yet … astronomy, like all sciences, is a field in which discoveries keep undermining theories. When scientists analyzed the water in comets, they discovered it wasn’t the same as the water in our oceans. Heavy water forms when deuterium (which has one proton and two neutrons) is present along with hydrogen (which has one proton and one neutron). And the water in comets is significantly heavier than the water in our oceans. So astronomers kept looking.
Three years ago, using the UH 2.2-m telescope on Mauna Kea, astronomers came across what could be the answer in the Asteroid Belt, that badlands between Mars and Jupiter where a gang of at least two hundred million asteroids orbits. Asteroids, first detected at the beginning of the nineteenth century, are a relatively new discovery in the world of astronomy; they are, in essence, big pockmarked chunks of rock, orphans from the solar system’s early days when most of the material in the sun’s proto-planetary disc was compressing to form Venus, Mercury, Saturn, Neptune and the other planets. Unlike comets, asteroids weren’t thought to contain water—until the astronomers using the UH telescope detected a new subclass of asteroids with large chunks of ice on their surfaces. Could a rogue asteroid or two be responsible for our oceans? UH is now lobbying NASA to send a spacecraft to the Asteroid Belt to take a sample of the ice and see whether it matches.
Is There Anybody Out There? Methane on Mars
With all of those planets and with all of that potential for water, the biggest question in astronomy can’t be far behind: Is there life out there? A recent discovery on Mars pushes that question too. In 2003, astronomers using the NASA Infrared telescope on Mauna Kea found methane on the red planet. “Amazing,” says Taft Armandroff, the director of the Keck telescopes. “I was blown away by this.” To understand why, you have to understand something of methane itself. It’s a transient compound that wouldn’t last longer than three hundred to six hundred years in Mars’ atmosphere; to be present, it has to be regenerated. And to regenerate methane, you need either a biological or geological process. In other words, you need some form of life or something akin to Mauna Kea itself. A Vulcan or a volcano, take your pick.
Astronomers took follow-up readings in 2006, found far less methane and concluded that three years earlier they’d stumbled on a burst in methane production. They are now doing further studies using the ultra-exact equipment at Keck. (Kudritzki points out that such a pattern of detection is classic on the mountain: Astronomers often make initial discoveries using the smaller telescopes, then refine their findings with the larger ones.) They’re also using the telescope to look for other molecules that might offer clues about the methane’s origins. Keck has already, says Armandroff, localized methane to certain sites on Mars—sites that are rich with water-bearing minerals—and will be able to pinpoint it further with adaptive optics. “If we can really localize it, then we can send spacecraft to probe the soil,” says Armandroff. “It’s so exciting that there could be some other life on Mars, even if it’s extraordinarily simple microbial life. That would, of course, be a first.”
The Huge Surprise: Dark Matter
For all of the answers found on Mauna Kea, new questions keep arising. “One of the exciting things about astronomy,” says Kudritzki, “is that you find things that surprise you. And you have many times an observed fact, but you don’t have an explanation.” In other words, it’s perfectly common to discover something and not have the faintest idea what it is. Such is the case with dark matter.
The first suggestions of its existence came with Fritz Zwicky in the 1930s and Vera Rubin in the 1960s. They looked at the huge velocities and centrifugal forces pushing and ripping at galaxies and wondered why the galaxies didn’t just come apart. Could it be, they theorized, that there was some hidden matter holding them together?
It was a controversial idea. If it were true, astronomers reasoned, then one way to prove it would be to look at the movement of light. Matter, Einstein had proved, warps light because everything with mass has a gravitational pull. When light approaches matter, it bends in response to that pull, an effect astronomers call “gravitational lensing.” They started looking for it.
At the same time, in the 1980s, astronomers at Mauna Kea’s Canada-France-Hawai‘i Telescope (CFHT) were using the telescope’s wide-field imaging camera to do a comprehensive deep survey of massive clusters of galaxies. And they found … gravitational lensing. They watched the way the light from background galaxies was deformed into a thin arc by a cluster of galaxies in the foreground, knowing it was impossible that the visible matter in those foreground galaxies had sufficient mass to bend light to such an extent. Looking at those stretched arcs, the astronomers realized that they were literally in the dark.
“What we know now is that when you look at a picture of a galaxy, what you see—the visible matter—is only 10 percent of the real matter in the universe,” says Kudritzki. “The other 90 percent is ‘dark matter.’ Here we are, doing astronomy over hundreds of years, very proud of our discoveries, and then we had to learn that we missed 90 percent. Dark matter is the dominant force that keeps the universe together. It’s everywhere.”
But what is it? Nobody has a clue.
“Dark matter is just an artificial term,” says Armandroff. “It’s matter that’s there that doesn’t really interact much with the baryonic matter we’re familiar with: protons, electrons, neutrons. There are theories that explain dark matter, but we don’t know what particle it is yet. That’s a major area of astronomy and physics right now. Could dark matter be neutrinos, subatomic particles that don’t interact with much? It doesn’t look like it. Keck is trying to get clues from the way the dark matter is distributed, but we can’t do it alone. It’s going to take particle physics, really. Astronomy and physics are intersecting very strongly right now.”
“Without astronomers, physicists wouldn’t have a job,” laughs Kudritzki. “We make the detections, and they build the accelerators and start the theories.”
The Great Force: Dark Energy
Dark matter is not the only vast mystery. Perhaps it should come as no surprise that the universe is full of “dark energy,” too. We can’t see it, we can’t feel it, but it’s “the biggest constituent of the universe,” says Armandroff. “And we didn’t know about it twelve years ago.”
The detection of dark energy traces to Edwin Hubble’s groundbreaking work in the 1920s that helped to prove the universe was expanding and galaxies were moving away from each other. The next questions were inevitable. If the energy from the Big Bang were still pushing the universe outward, astronomers wondered, how would that expansion proceed in the future as the energy waned? Would it slow down? Stop? Would the universe eventually begin to contract?
To answer those questions, astronomers sought to determine the rate at which the universe was expanding. They discovered they could measure the rate of expansion by finding “fixed points”: a class of supernovae that had an intrinsic, unchanging brightness. They dubbed these supernovae “standard candles” and at the end of 1990s initiated a systematic search for them using, on Mauna Kea, the low-resolution spectrograph at Keck and the wide-field telescopes of Subaru and CFHT, as well as other telescopes around the world and the Hubble Space Telescope and other telescopes around the world.
What they found astonished everyone. The expansion of the universe was not slowing down, but rather accelerating. “It’s completely counterintuitive and one of the most exciting detections in modern astronomy,” says Kudritzki. “There must be a source of energy in the universe that’s driving this. We call it ‘dark’ because we can’t see it and we don’t know what’s behind it. The basic idea is that there must be elementary particles of energy in the universe that form the basis of it, but we don’t know what they are.”
“It’s incredibly exciting,” says Armandroff, “and really mind-boggling. Something has to drive this. You can’t get an acceleration from nothing. The biggest skeptics say it’s an artifice. No one can put their hands on it or measure it in any other way except for these astronomy experiments that show these accelerations. But it has since been confirmed with objects other than supernovae. This is a force that’s all around us. One of Einstein’s pioneering concepts is that mass and energy are equivalent. And astronomers have a pretty good idea of how much mass there is in the universe: stars, planets, gases. And all mass pales in comparison to the amount of dark energy. That’s amazing!”
The next telescope planned for Mauna Kea will stand thirty meters high—three times the size of the largest telescope on the mountain now, Keck. It will provide astronomers with more light and more definition, two vital assets that will allow scientists to scrutinize everything they’re looking at now with far more precision: the planets, the stars, the dust clouds, the galaxies, the very distant universe. Everything will be clearer, closer, in sharper relief—less mysterious, more known. And given what’s already been detected on Mauna Kea, Kudritzki marvels at the prospect not just of what we’ll learn about other worlds, but of what we’ll learn about our own.
“We are making detections that indicate that our knowledge about not only the universe but the structure and nature of matter itself is incomplete,” he says and then he adds—half-shrugging, half-laughing and utterly enthused—“we’ve only seen the tip of the iceberg” … if that.